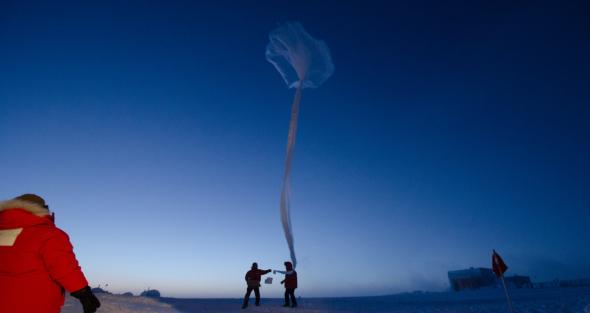
The Cambridge Zero Research Symposia launched a new series for Michaelmas Term 2021 with the theme of Climate Science on the 6th of October 2021. Bringing together academics and early career researchers, the afternoon showcased the latest research taking place in this field across the University of Cambridge and British Antarctic Survey. It highlighted the multi-pronged effort required to reach net zero. The fundamental scientific models spanned the physical environment, from the atmosphere to the oceans and ice sheet loss.
This blog provides a summary of what each speaker shared with the audience, starting Professor Eric Wolff (Earth Sciences), and followed by Dr Alex Archibald (Chemistry), Dr Alison Ming (DAMTP), Dr Emma Boland (British Antarctic Survey), Xiaoqing Chen (Earth Sciences), Sam Lewin (DAMTP) and Arianna Cox (DAMTP).
Professor Eric Wolff "What can the past tell us about future ice sheet loss and sea level rise?"
Department of Earth Sciences
“You have to know the past to understand the present,” Carl Sagan
Context is vital in spotting trends and anomalies. It is through the perspective of setting the present against the backdrop of the past that we can help project future conditions. Professor Eric Wolff opened the session with the first fact that global sea level has already risen by 20cm since the beginning of the Industrial Period. Future sea-level rise will depend on the emissions trajectories we choose to follow. Emissions scenarios used in climate models (IPCC Sixth Assessment Report, 2021) describe how greenhouse gas emissions could evolve, providing a set of possible future trajectories. In each of the scenarios, there is significant uncertainty in the expected sea-level rise, and as such, it can vary between less than 1m right up to 10m by 2300. Part of the uncertainty is related to the possible inherent instability of the Antarctic ice sheet.
In the past, polar regions were sometimes warmer than they are today, and this raises the question as to whether their past behaviour may constrain future change. Historical temperature records from ice cores in Antarctica and Greenland for the past 150,000 years show that the last 10,000 years (Holocene period) experienced relatively stable temperatures until the last few decades, as shown in Image 1. Prior to that, the Glacial period (120,000 to 11,500 years ago from today), during which a large part of the earth's surface was covered with ice, brought sea levels 120m lower than current levels.
Image 1: Temperatures of Greenland and Antarctica over the past 150 thousand years (E. Wolff)
Analysing the global temperatures of 130,000 years ago shows that during the Last Interglacial (period of milder climate before the last glacial period) Antarctica and Greenland were at elevated temperature levels compared to today’s temperatures. This is attributed to changes in the Earth’s orbit around the Sun and changes in ocean circulation, and not to high levels of carbon dioxide in the atmosphere, as is the case today.
Several studies have estimated the Last Interglacial (130k to 115k years ago) sea level rise (using data from climate archives such as corals) compared to present levels, including:
6-9m – Dutton et al. (2015)
5-10m – IPCC, 6th Assessment Report (2021)
Out of this, 1m rise will have come from thermal expansion of the ocean and the melting mountain glaciers, and 2m rise will likely have occurred due to ice sheet melt from Greenland. This implies substantial loss from the Antarctic ice sheets.
The future of the West Antarctic Ice Sheet (WAIS), the segment of the continental ice sheet that covers West Antarctica, is crucial for future sea levels. The stability of WAIS in the past during the Last Interglacial period shows it as a predictor for the year 2100. If the WAIS retreated at that time, then it has the potential to also retreat by 2100 and beyond.
Prof Wolff presented data from a 651 metre-deep ice core which reached the bottom of the ice sheet at SkyTrain Ice Rise on the edge of WAIS. This was collected in 42 days of drilling in the austral summer of 2018-19. The ice was processed by melting a section of it at a rate of a few centimetres per minute, with the released air and melted water transferred into a chemistry lab for analysis.
The first exciting result is that the data show that the core did indeed reach into the last interglacial at 125,000 years, and provides a record of climate over that period. While the data are still being carefully assessed, one result is that the concentration of sea salt did not increase in the way we would have expected if the nearby Ronne ice Shelf had collapsed. This may therefore be good news, suggesting that the ice shelf is more stable than some have feared. However, it puts the spotlight on other areas of Antarctica and Greenland, as the sea level rise still has to be explained.
Dr Alex Archibald "Earth system models and their use in solutions to the climate crisis"
Department of Chemistry
The Earth System is complex, and incorporates many interconnected processes, as shown in Image 2. This includes the physical climate system (ocean, land and the atmosphere) as well as ecosystems (vegetation growth and marine organisms). The chemistry from the ecosystems, such as plants or trees, release biogenic volatile organic compounds (hydrocarbons produced by plants in various tissues above and below ground). These can go on to become aerosols, which in turn affect other processes, for example clouds that feedback into the physical climate.
Image 2: The Earth System (T. Kuhlbrodt). Adapted from Earth System Modelling in the UK | Weather and Climate @ Reading
Modelling these physical processes provides a pathway to predict potential climate outcomes. The systems are compartmentalised into subcomponents of the models.
Prof Alex Archibald’s research group works in partnership with the UK Met Office, using the Unified Model. With approximately two million lines of computer code, this model is used for short- and medium-term weather predictions, as well as climate projections for the year 2100 and beyond.
In a similar fashion, the research group is developing the chemistry part of the Earth System model, known as the United Kingdom Chemistry and Aerosols (UKCA) model. This model interacts with the other systems’ models which sit in Earth System’s model matrix (ESM), for example the atmosphere, radiative properties in the atmosphere and oceans. ESM models identify the compounds heating the atmosphere.
Dr Alison Ming, "When the heart of the stratosphere skips a beat"
Department of Applied Mathematics and Theoretical Physics
Dr Alison Ming’s research explores the processes in the stratosphere (approximately 10km-30km above the Earth’s surface), and in particular the phenomena of the Quasi-Biennial Oscillation (QBO), often called the “beating heart of the stratosphere”.
It was in 2016 that the QBO, which usually follows a regular oscillation pattern, missed a beat. Something which had not been previously observed, and which Alison’s research has worked towards quantifying. The procedure examines the data of the earth’s equatorial zonal winds, which includes the change in pressure with height from the Earth’s surface over a period of time.
Image 3: Earth’s atmospheric layers (R. Rehman)
Zonal winds circulate in a tightly constrained area above the earth’s surface - between 17-36km and latitudes of between North-South of 30o – parallel to the equator. In general terms, the two winds (a westward and an eastward wind) oscillate and reverse over 28 months. The winds propagate downwards in a doughnut shape, until they dissipate at the bottom of the stratosphere. This is the Quasi-Biennial Oscillation, and it is the regular movement of the winds towards the bottom of the stratosphere over the 28-month period, which gives it the descriptor of the “beating heart of the stratosphere”.
The variability of the lower stratosphere is therefore timely and thus reliable and provides an excellent means for seasonal predictability. It can affect the concentration of the gasses in the stratosphere, which influence the atmosphere at the Earth’s surface. The system is interconnected, and can impact atmospheric chemistry, radiative heating, ozone, carbon dioxide, water vapour, waves, atmospheric transport (movement of compounds or particles from one region to another via the atmosphere) and clouds.
The lower stratosphere receives most of its air from the troposphere (see Image 3), which tends to be wet. The temperatures close to the boundary between the troposphere and stratosphere control the amount of water vapour which moves into the lower stratosphere. At lower temperatures, “more water vapour is freeze-dried out of the air before it reaches the stratosphere”, more water vapour is removed, and thus drier air enters the stratosphere. At higher temperatures, more moist air enters the stratosphere.
In 2016, a Westward jet in the zonal winds appeared in the lower stratosphere, in the Eastward Phase of the Quasi Biennial Oscillation. Its presence raised the question of its effect on the temperature, and the radiative effect. Alison Ming’s study found a large change in the temperature of the lower stratosphere of around 3oC. The skipped beat showed an unusually large change in ozone.
Dr Emma Boland "The impact of aerosol forcing strength on ocean heat uptake"
British Antarctic Survey
Aerosols in the atmosphere can have a direct effects on the earth’s energy balance by the scattering, absorption or re-radiation of the sun’s incoming radiation, as well as indirect effects including cloud interactions. Overall, increasing aerosols in the atmosphere results in overall cooling. These are complex processes to model, and earth system models vary widely in their representation of aerosol processes.
The upward trend of the global mean surface temperature in the 2000s slowed down, despite the CO2 levels continuing to rise. This is referred to as the “global warming hiatus”. It was established that the hiatus was partly associated with an increase in heat uptake by the deep oceans, especially in the Pacific. As a part of the “Securing Multidisciplinary UndeRstanding and Prediction of Hiatus and Surge events” (SMURPHS) project, Dr Emma Boland’s work examined how changing the amount of anthropogenic aerosols in the atmosphere affects multi-decadal trends of global ocean heat content in the Met Office’s Hadley Centre global climate model.
Image 4: Ocean Heat Content from 25 model ensemble members forced by five different anthropogenic aerosol time series (colours, produced by scaling the historical baseline case [1.0]), for the global ocean, and the four major ocean basins. (E. Boland)
Her study showed that the centennial scale linear response and decadal variability of global ocean heat content (OHC) is significantly sensitive to aerosol forcing strength, but inter-annual variability in OHC is relatively insensitive. The impact of the aerosol forcing factor varies widely by location - in the North Atlantic internal variability dominates, whereas Ocean Heat Uptake in regions such as the tropics and Eastern Pacific is linearly dependent on aerosol forcing strength.
Xiaoqing Chen "Time-lapse volumetric seismic imaging of water masses at a major oceanic front"
Department of Earth Sciences
Oceanic measurements are primarily of temperatures and salinity (thermohaline information). These are taken at different depths in the ocean using multiple types of oceanographic measurement equipment, for example by transmitting observations by copper coil back to the surface. To increase resolution, seismic oceanography is used to complement such measurements. Building on a well-established geophysical technique, this approach transmits acoustic waves into the ocean, and the reflected signals are picked up with hydrophones (a microphone which detects sound waves under water) on the sea surface. The acoustic waves bounce back at boundary points of changes in temperatures and salinity, which help to create subsea maps of these boundaries.
Image 5: Diapycnal mixing diffusivity calculated using spectral analysis at a major oceanic front in Brazil-Malvinas Confluence. From blue to red: low diffusivity (weak mixing process) to high diffusivity (enhanced mixing process) (X. Chen)
It is known that the technique shows the acoustic wave speed in the ocean depends 80% on the temperature and 20% on the salinity. It can also have a temperature change resolution of 0.3oC and is able to cover sea surface areas of up to hundreds of kilometres.
The global thermophile circulations provide critical oxygen and nutrient transportation in the oceans, but the mechanisms are not fully understood. Emma Chen’s research has focussed on the Brazilian Malvinas front, a dynamic region of water off the coast of South America. It brings the warm and salt water of the Brazilian current towards the south, and Malvinas current brings the cold and fresh water to the north.
The spectral analysis allows internal waves in different water layers to be tracked, and the energies calculated. The seismic images allow analysis of mixing of waters with different temperatures and salinity from two sides, as well as particle displacement.
Sam Lewin "Improving models of ocean turbulence with data-driven methods"
Department of Applied Mathematics and Theoretical Physics
Ocean turbulence provides the means for vertical mixing of heat, carbon and nutrients in the ocean. It brings the cold water from the deeper regions in the oceans to the surface. The energy for the turbulence is provided by the internal gravity waves at the interface of water layers of different densities.
Sam Lewin’s work concentrates on two internal-driven wave mixing mechanisms. The first is the investigation of individual wave breaking processes. The second is building practical models which calculate mixing rates from oceanographic datasets. The datasets are gathered using probes designed to measure water shear fluctuations. Microstructure profilers are sent down through the water column to depths of up to 6km.
The simulations are trained on where the answer is known, and take into consideration the uncertainties which arise, such as simplification of properties. The simulations are then related to observations by sampling individual vertical columns. The machine learning model uses probabilistic neural networks (PNN), which take the actual shear measurements and density gradients from the microstructure profilers to provide the dissipation. It also provides uncertainty estimates by means of feeding the same input into the network multiple times, and getting a distribution of outputs. The model thus provides the mean and variance, which are learnt by the network.
The performance of the model can be evaluated by the frequency distributions.
Arianna Cox "Methane removal using photocatalysis"
Department of Applied Mathematics and Theoretical Physics
The recent IPCC report suggests 30-50% of the current rise in temperatures is due to methane. The major contributors to methane release are: the digestive systems of cows, rice cultivation, wetlands, landfill, thawing permafrost, leaks from gas fields and coal mines and termites. Current methods for methane removal include combustion or thermocatalysis. Thermocatalysis is an efficient way for removal, and as the oxidation of methane is exothermic, it can be used to provide electricity. However, thermocatalysis requires specialised equipment, and the catalyst deactivates fast in high temperatures.
Arianna Cox’s research focus on photocatalysis for methane removal is driven by the need for little equipment, its effectiveness at low concentrations and temperatures and the use of inexpensive catalysts such as zinc oxide, which is already widely manufactured. The catalyst itself can be placed outdoors to react with UV light.
The two-step pathway of the reaction of methane and oxygen with the photocatalyst zinc oxide results in an intermediary of formaldehyde (HCHO) and water. The formaldehyde then further reacts with oxygen to produce carbon dioxide and water. Although carbon dioxide is a greenhouse gas, over a 20-year period methane is 80 times more efficient at trapping radiation.
Image 6: Model approach (A. Cox)
A model (Image 6) was used to estimate how much methane could be removed. In the first instance, this was simplified to examine the methane concentration (100ppm to 10,000ppm) over time using zinc oxide. The methane decayed exponentially. Arianna Cox’s work incorporated three models.
Potential future applications and locations of this approach for methane removal include:
- Walls of cow sheds
- Around leaking gas fields
- On the top of cars or trains
- Coating skyscrapers or roofs
- Solar updraft towers
Original Cambridge Zero publication.
Image: Launching an ozone sonde balloon. Credits: National Oceanic and Atmospheric Administration Photo Library, Unsplash
Raheela Rehman is is the Energy Interdisciplinary Research Centre (Energy IRC) Project Officer at the University of Cambridge. The Energy IRC plays a central role in supporting energy research across the University. It brings together the collective deep knowledge and research expertise of the University — from science and engineering through to policy, social sciences and humanities — to tackle the grand technical and intellectual challenges that must be overcome to achieve a global transition to a zero-carbon future. |