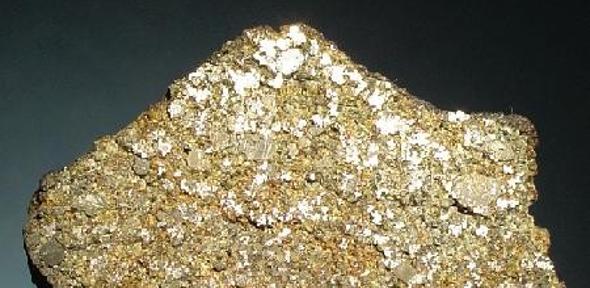
A team from the University of Cambridge, working with colleagues from Austria, found a new way to make a possible replacement for rare-earth magnets: tetrataenite, a ‘cosmic magnet’ that takes millions of years to develop naturally in meteorites. Previous attempts to make tetrataenite in the laboratory have relied on impractical, extreme methods. But the addition of a common element – phosphorus – could mean that it’s possible to make tetrataenite artificially and at scale, without any specialised treatment or expensive techniques.
The results are reported in the journal Advanced Science. A patent application on the technology has been filed by Cambridge Enterprise, the University’s commercialisation arm, and the Austrian Academy of Sciences.
High-performance magnets are a vital technology for building a zero-carbon economy, and the best permanent magnets currently available contain rare earth elements. Despite their name, rare earths are plentiful in Earth’s crust. However, China has a near monopoly on global production: in 2017, 81% of rare earths worldwide were sourced from China. Other countries, such as Australia, also mine these elements, but as geopolitical tensions with China increase, there are concerns that rare earth supply could be at risk.
"Rare earth deposits exist elsewhere, but the mining operations are highly disruptive: you have to extract a huge amount of material to get a small volume of rare earths.
Between the environmental impacts, and the heavy reliance on China, there’s been an urgent search for alternative materials that do not require rare earths," Professor Lindsay Greer, Department of Materials Science & Metallurgy
Tetrataenite, an iron-nickel alloy with a particular ordered atomic structure, is one of the most promising of those alternatives. Tetrataenite forms over millions of years as a meteorite slowly cools, giving the iron and nickel atoms enough time to order themselves into a particular stacking sequence within the crystalline structure, ultimately resulting in a material with magnetic properties approaching those of rare-earth magnets.
At first glance, the diffraction pattern of tetrataenite looks like that of the structure expected for iron-nickel alloys, namely a disordered crystal not of interest as a high-performance magnet. It took Ivanov’s closer look to identify the tetrataenite, but even so, Greer says it’s strange that no one noticed it before.
The researchers say that phosphorus, which is present in meteorites, allows the iron and nickel atoms to move faster, enabling them to form the necessary ordered stacking without waiting for millions of years. By mixing iron, nickel and phosphorus in the right quantities, they were able to speed up tetrataenite formation by between 11 and 15 orders of magnitude, such that it forms over a few seconds in simple casting.
Image credit: Rob Lavinsky, iRocks.com