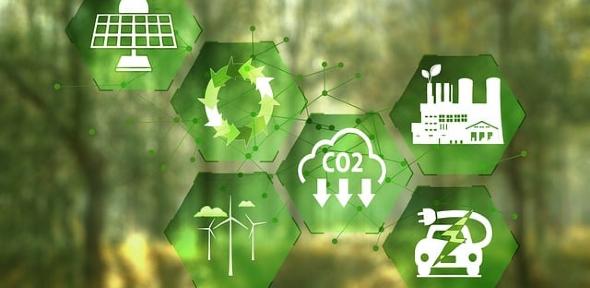
A number of Cambridge engineers have secured funding for various research projects focused on decarbonisation and low carbon energy.
Original Department of Engineering article
We at the Energy Interdisciplinary Research Centre (IRC), with Higher Education Innovation Funding (HEIF), were pleased to support the latest round of Small Grants for impact and knowledge exchange for the interdisciplinary teams working across a variety of topics.
These topics include:
- Computational studies of CO2 storage feasibility in underground rock formations
- Under what conditions can green hydrogen be competitive? Lessons learnt from China’s pilots
- Fabricating ultra-low-power non-volatile resistive-random-access (RRAM) memories using a novel iontronic-based material design
- An investigation of how to handle the intermittency of renewable power in electrified chemical processes
Field and computational studies of CO2 storage feasibility in an underground rock formation
Illustration showing the carbon capture and storage process, and rock cut. Credit: Qian Cheng.
PhD student Qian Cheng, from the Department of Engineering’s Geotechnical and Environmental Research Group, has worked with Dr Dongfang Liang, Professor of Hydrodynamics, and Jiawei Lu, from the Cavendish Laboratory: Department of Physics, to investigate technological advances for the optimisation of carbon capture and storage (CCS) in geological formations.
CO2 captured from cement and steel production, for example, can be stored underground in porous rock formations known as deep saline aquifers, which are initially saturated by formation water (brine). As carbon is injected, the defending brine is displaced, and CO2 can be stored in these rocks for thousands of years, if not permanently.
Since September 2022, there continue to be 196 carbon storage projects in operation or active development, pumping 42.5 megatons of carbon into subsurface aquifers annually.
But with more countries expected to pour resources into such projects, resulting in an inevitable increase in the annual carbon injection amount, efforts to fully understand how an injected CO2 plume travels inside the aquifer is of the utmost importance, say researchers.
“The complex fluid dynamics involve preferential flow paths that often lead to inefficient use of storage areas, for example a CO2 plume that only occupies a fraction of the available storage space,” said Qian Cheng. “Thanks partly to the IRC Small Grants award, we have built a two-dimensional model of a typical carbon injection site to investigate whether suppressing such flow paths can maximise CO2 injection efficiency.”
This two-dimensional model, say the research team, is an essential step towards a full-fidelity three-dimensional simulation, capable of conducting fluid simulations to identify the most important factors influencing a typical carbon injection site’s storage efficiency.
“CO2 is stored in these geological formations mainly through four types of mechanisms: structural, capillary, dissolution and mineral trappings,” said Professor Liang. “We can thoroughly investigate these four types of trapping mechanisms with the new high-fidelity model and the immense computational power provided by the University’s high performance computing service. This is part of ongoing research.”
Field trip: green hydrogen development in China and lessons learnt for the UK hydrogen industry
Illustration showing a green hydrogen production concept. Credit: scharfsinn86 – stock.adobe.com.
PhD student Hanzhe Xing, from the Department of Engineering’s Energy Group and the University’s Energy Policy Research Group (EPRG), conducted a field trip to China to evaluate the potential of green hydrogen and the future business model of its international trade, using China as a case study.
Green hydrogen is hydrogen produced by a process of electrolysis, using renewable energy. The field trip incentivised Hanzhe to conduct an independent research project on the international green hydrogen product supply chain, which he conducted in collaboration with the China Hydrogen Energy Alliance Research Institute.
The aim is to formulate new research ideas of how to optimise green hydrogen production in the UK, using dedicated electricity with a mix of electrolysers with different ramp-up rates. Electrolysers help produce low-emission hydrogen from renewable or nuclear electricity and use electricity to separate water into hydrogen and oxygen.
Hanzhe said: “During the field trip, I visited the Hydrogen and Fuel Cell Industry Research Institute of the National Energy Investment Group and two green hydrogen factories under construction. It turns out that the technology development of the life cycles of electrolysers has reduced the cost of making green hydrogen, especially when using cheap, dedicated electricity.”
He added: “The IRC Small Grants award has supported interdisciplinary research in energy in three ways: through sharing knowledge on the production and global supply chain of green hydrogen in China; by adding the value of carbon quantitively to current green hydrogen evaluation research, as well as to the business model for green hydrogen; and finally, using deep reinforcement learning and adding long-term time series forecast technologies to traditional models of green hydrogen production, promoting the accuracy of the model.”
A novel iontronic-based material design for fabricating ultra-low-power non-volatile resistive-random-access (RRAM) memories
Dr Bakhit pictured looking at a two inch silicon (Si)/200 nm Si wafer, sputter-coated first with titanium nitride (TiN), followed by a complex oxide thin film, before top electrode patterning. Credit: Kasia Surowiecka.
Research Fellow Dr Babak Bakhit, from the Department of Engineering’s Electronic Devices and Materials Group, has worked with Andrew Flewitt, Professor of Electronic Engineering, and Professor Judith Driscoll, from the Department of Materials Science and Metallurgy, to discover novel iontronic-based functional oxide thin films to tackle the huge energy consumption of the current data storage and computing technologies.
In the today’s data-hungry world, data storage and computing technologies consume a huge share of the world’s electricity and have already become one of the main global issues accelerating the climate crisis. For example, training one single artificial intelligence (AI) model can be more than five times worse for the Earth than driving one car. The pace of digital innovation in AI chips, together with cloud storage and computation, security, electric vehicles, telecommunication, robotics, Internet of Things, wearable devices, etc. critically depends on expanding the power of storing and processing data.
Intensive data processing poses serious difficulties for conventional computers. A computer’s computation and storage units are physically separated, and most energy and time are consumed in the frequent data movements between these two units. Thus, to meet the net zero objectives, high-performance, energy-efficient memory and computing devices are urgently required.
Since 3-terminal semiconductor-based transistors have already reached their quantum-mechanical and fabrication limits and are not adequately efficient for advanced memory and computing technologies, 2-terminal easy-to-fabricate insulating-based devices – with fundamentally different materials and device physics – are gaining considerable attention as being the next generation of high-throughput, energy-efficient data storage units. In this context, emerging oxide-based non-volatile memories such as ferroelectric and resistive random-access memories, which retain stored information without requiring external power, are being globally explored.
“Despite extensive study in the field, these emerging technologies are still in the development phase and need significant advances to achieve the requirements for the mass production of both processor and memory units,” said Dr Bakhit. “This will require revolutionary innovations in their material design and fabrication.”
“Supported by the Swedish Research Council, the IRC Small Grants and CAPE BlueSky Research Awards, I am developing asymmetrically defective Hafnium oxide (HfO2)-based complex oxide thin films that have shown high promises for both memory and neuromorphic computing technologies. These devices, that their switching performance is mainly controlled through modulating the barrier height at their interfaces, are ultra-low current devices with excellent cycle-to-cycle and device-to-device uniformity; very high retention; adequately high cyclic endurance; and multilevel resistance states, all of which are essential for neuromorphic computing. They also show ferroelectric responses, again with ultra-low switching currents.”
Dr Bakhit added: “These advanced oxide thin layers are fabricated via only one-step co-sputtering, which is an environmentally friendly vacuum-based fabrication technique, on sputter-deposited titanium nitride (TiN) layers that serve as bottom conductive electrodes (all in one deposition chamber and without breaking the vacuum).”
This fundamental research will result in a new class of emerging devices with a novel material design, which is currently lacking in the community. The aim is for world-scale impacts in semiconductor technologies that along with quantum computing, artificial intelligence, bioengineering, and telecoms, are envisaged as one of the five ‘technologies of tomorrow’ in the UK. The results of this project will strongly contribute to establishing advanced novel oxides for substantial data storage and industrial-scale neuromorphic computing devices in the future.
Investigation of industry perspectives on handling the intermittency of renewable power in electrified chemical processes
George Fulham, a PhD student from the Department of Chemical Engineering and Biotechnology, is investigating the utilisation of renewable power for the synthesis of carbon-neutral chemicals and fuels, known as Power-to-X.
The work builds upon prior investigations alongside Dr Paul Hodgson, Technical Lead on the Aviation Impact Accelerator (AIA) and Professor Rob Miller, Director of the Whittle Laboratory, University of Cambridge.
Insights from George’s research on these power-to-X fuels have been used to inform the AIA’s modelling of fuel production, and in addition, has added knowledge of the systems-wide challenges.
The AIA aims to accelerate the journey towards carbon-neutral aviation by developing evidence-based models and insights to support policy and direct further technical developments.
George said: “I have had numerous conversations with those involved in researching implementing large-scale Power-to-X projects – specifically the conversion of CO2 to methanol.
“Over the course of these conversations, it emerged that the implications and challenges of renewable power intermittency – i.e. the variation in power according to weather variations – have not been fully considered or deemed an external problem to be obviated in due course through wholesale decarbonisation of the grid system, which is unlikely to materialise.
“Specifically, the time variability of renewable power means that chemical processes can no longer be run in a steady, continuous mode, as has historically been the case, meaning that we must consider dynamic operation modes.
“However, the Power-to-X processes all rely heavily upon suitable catalysts to facilitate the chemical synthesis, for which the issue of catalyst stability and deactivation is a pressing concern in need of further research. If catalysts undergo severe degradation, especially when operated dynamically, the viability of the CO2 conversion process suffers severely. Therefore, a better understanding of operational limits of catalysts is essential.
“Therefore, alongside systems-wide modelling of dynamic plant operation, I have experimentally monitored changes to the catalysts over long periods up to 50 hours, when exposed to gas atmospheres of CO2, H2, and H2O – similar to those experienced when the catalysts support CO2 conversion to methanol.
“The experiments have yielded valuable insights around the reduction and oxidation behaviour of different candidate catalysts to probe reasons for their degradation or stability under different conditions. A portion of the work has been published in the Chemical Engineering Journal, and has been presented at the UK Catalysis Conference earlier this year.”
Image credit: geralt